Abstract
Asbestos is a general name given to a group of carcinogenic and genotoxic fibrous silicate minerals that have been of commercial values over the years. They include chrysolite, amosite, crocidolite, tremolite, actinolite and anthrophylite. Because of their special properties such as flexibility, tensile length, heat stability and resistivity to chemical, electrical, thermal and biological attacks, asbestos fibres have been used as components of several construction, building and automotive materials. This materials when disturbed, during home redecoration, demolition, renovation and automobiles repairs, release asbestos fibres into the atmosphere leading to both residential and occupational exposure. Measured concentration of fibres has been found to be higher in urban areas (especially those in close proximity with asbestos industries which may include mining and demolition sites) than in rural areas. Several studies have linked asbestos exposure to several serious health challenges such as lung cancer, mesothelioma, asbestosis, and other respiratory diseases. Although the main mechanisms through which asbestos can lead to diseases are not fully understood, three hypotheses have been made. These include alterations at the chromosomal level, activation of oncogenes, loss of tumour suppressor genes, alterations in cellular signal transduction pathways, generation of reactive oxygen and nitrogen species, and direct mechanical damage to cells from asbestos fibres. This article reviews briefly the ways by which the general public (occupational and non-occupational) can be exposed to asbestos and its most recent pathogenic mechanisms leading to malignant and non-malignant disease conditions.
Keywords
License
This is an open access article distributed under the Creative Commons Attribution License which permits unrestricted use, distribution, and reproduction in any medium, provided the original work is properly cited.
Article Type: Research Article
EUR J ENV PUBLIC HLT, Volume 3, Issue 2, 2019, Article No: em0030
https://doi.org/10.29333/ejeph/5906
Publication date: 02 Sep 2019
Article Views: 5368
Article Downloads: 3866
Open Access HTML Content References How to cite this articleHTML Content
INTRODUCTION
Asbestos is the generic name given to a commercially and legally defined group of six naturally occurring fibrous silicate minerals that have current or historical commercial usefulness. They are used in products such as roofing shingles, water supply lines, fire blankets and insulation materials, as well as clutches and brake linings, gaskets and pads for automobiles, due to their extraordinary tensile strength, poor heat conduction and relative resistance to chemical attack (ATSDR, 2016; WHO, 2018).
Currently, about 125 million people in the world are exposed to asbestos at the workplace which has been linked to the estimated global deaths of about 107000 yearly (IARC 2012; WHO, 2018). WHO (2014) reported that the burden of asbestos-related diseases is still rising, even in countries that banned the use of asbestos in the early 1990s because stopping the use of asbestos resulted in a decrease in the number of asbestos-related deaths only after a number of decades due to its long latency periods.
Two main types of asbestos fibres are the serpentine; which member includes only chrysotile (also called the blue asbestos), and the amphibole; which members include Amosite, crocidolite (also called blue asbestos), tremolite, actinolite and anthophyllite – with chrysotile being the most commonly commercially used form of asbestos (WHO, 2014). However, the amphiboles composing of brittle, rod- or needle-shaped fibers are more hazardous than chrysotile when inhaled or ingested (WHO, 2014). According to HSE (2018), inhalation of asbestos fibres can cause a number of serious diseases most of which affect the lungs or pleura (the external lining of the lung); these include a number of forms of cancer and chronic conditions such as asbestosis and pleural thickening (mesothelioma). This article briefly reviews the various routes of exposure of the general public (occupational and non-occupational) to asbestos and its associated disease conditions.
COMPOSITION AND CHARACTERISTICS OF ASBESTOS
The amphibole and serpentine minerals occur both as asbestiform (fibrous) and nonasbestiform (massive) varieties of identical chemical composition. Chrysotile is a hydrated magnesium with individual fibrils having a size of 20–40 nm and when crushed, chrysotile ore produces fiber bundles consisting of variable numbers of aggregated individual fibrils – which have varying lengths that may exceed 100 mm (Nagoya, 2009).
Typically, chrysotile fibers exhibit a curved, curly, or wavy morphology that influences the interceptive deposition of chrysotile fibers, which in turn, affects the depth of penetration into the lower respiratory tract. The amphiboles are with a wide range of cation substitutions within the silicate backbone of the crystal structure. The predicted formulas of the asbestiforms (of both serpentine and amphiboles) are shown in Table 1.
Table 1. Chemical Compositions and Characteristics of Asbestos Fibres
Source: Nagoya (2009) |
HUMAN EXPOSURE
According to IARC (2012), inhalation and ingestion are the primary routes of exposure to asbestos. Dermal contact is not considered a primary source, although it may lead to secondary exposure to fibres, via ingestion or inhalation. The degree of penetration in the lungs is determined by the fibres diameter, with thin fibres having the greatest potential for deep lung deposition (NTP, 2005). Human exposure to asbestos can occur when asbestos-containing material (man-made or natural) is disturbed releasing asbestos fibers into the air (ATSDR, 2016). This could mean that asbestos embedded or contained in undisturbed solid materials presents a negligible risk of exposure.
Exposure of the General Population
Inhalation of asbestos fibres from outdoor air, and to a lesser degree in indoor air, is the primary route for the non-smoking general population. Exposure may also occur via ingestion of drinking-water, which has been contaminated with asbestos through erosion of natural deposits, erosion of asbestos-containing wastes sites, corrosion of asbestos-containing cement pipes, or filtering through asbestos-containing filters. Families of asbestos-workers may be exposed via contact with fibres carried home on hair or on clothing (IARC, 2012). Studies have shown that chrysolite is the predominantly detected asbestos in outdoor air, with higher measured concentration (10-fold higher) in urban locations, and 1000 times higher in close proximity to industrial sources of exposure (including asbestos mine or factory, demolition site, or improperly protected asbestos-containing waste sites) than in rural locations (with typical concentration of 10 fibres/m3) (ATSDR, 2001). In indoor air (e.g. in homes, schools, and other buildings), measured concentrations of asbestos are in the range of 30-6000f/m3.
Occupational Exposure
Globally each year, an estimated 125million people are occupationally exposed to asbestos (WHO, 2006). This exposure (by inhalation, and to a lesser extent by ingestion) occurs in the mining and milling of asbestos, the manufacturing or use of products containing asbestos, construction, automotive industry and the asbestos-abatement industry (IARC, 2012). Despite the evidence by OSHA and MSHA indicating a reduction in occupational exposures in the USA over the past several decades, report by Rice and Heineman (2003) indicated that the nature of occupational exposures to asbestos has changes, with maintenance activities and remediation of buildings that contain asbestos being the current occupational exposures. It is worthwhile to suggest that, since asbestos is also contained in materials such as vehicle parts, textiles, plastics and other minerals contaminated with asbestos (e.g. talc), workers in these industries are in higher risk of exposure.
PATHOGENESIS OF ASBESTOS
NIOSH (2011a) enumerated fibres size, bio-persistence, chemical composition, and particle surface characteristics as the main determinants of asbestos toxicity. ATSDR (2016) elucidated that the presence of asbestos fibres in the lungs sets off a variety of responses leading to inflammation, cell and tissue damage, which can lead to malignant and nonmalignant diseases. Extensive epidemiological studies have established associations between asbestos exposures, lung cancer, mesothelioma and other respiratory diseases (IARC, 2012). The toxic effects of asbestos inhalation depend on the cumulative dose, the time of initial exposure, and both the physical and chemical properties common to the different asbestos fibres (Kamp, 2009). Upon exposure, amphibole fibres – which are uniform in distribution and arrangement, align parallel to the axis of airflow and typically deposit in alveolar ducts; whereas serpentine fibres, which are heterogeneous in arrangement, have a mixed flow pattern and will usually deposit in airway bifurcations (Steele, 2011). Fibres that land in ciliated portions of the airways are typically removed via the mucociliary escalator and do not result in lung injury (IARC, 2016). Amphiboles asbestos (particularly amosite and crocidolite) are thought to be more harmful to human health than serpentine asbestos (NIH, 2009).
The precise mechanisms to explain asbestos fibre toxicity are poorly understood and may be a consequence of the differential deposition pattern, surface reactivity, or biodurability of these fibres. Three pathogenic pathways are hypothesized to induce asbestos-related diseases. These include inflammation, apoptosis, and oxidative stress. Shortly after contact with asbestos fibres, the first cells that come into contact with inhaled asbestos fibres (epithelial cells lining the airways and alveoli) are directly injured (Solbes and Harper, 2018). This injury has been shown to occur through both cytolytic and non-cytolytic mechanisms in humans. Increased permeability of the epithelium can lead to fibrosis through increased movement of inflammatory mediators from the alveoli into the interstitium and by causing collapse of small airways and alveolar ducts (Wright et al., 1992). In the alveolar duct, alveolar macrophages phagocytise the fibres, after which they are transported along a microtubule network to the perinuclear region inducing further inflammation and damage (Cole et al., 1991).
In addition to direct mechanical injury, generation of reactive oxygen species (ROS) through various mechanisms that induce further damage has also been reported prior to asbestos exposure. One mechanism is a direct effect from the asbestos fibres themselves; where iron on the asbestos fibres serves as a catalyst for the Fenton reaction, which generates a highly toxic hydroxyl radical from hydrogen peroxide (Nagoya, 2009). Besides damage to cells, ROS can cause DNA strand breakage in mesothelial cells, which can increase the risk of developing malignancy (Sekido, 2013). There is also evidence that reactive nitrogen species (RNS), in addition to ROS, play a role in asbestos-related lung injury. Inhaled asbestos fibres can induce the activity of inducible nitric oxide synthase in alveolar macrophages, A549 cells (a cell line derived from a human lung cancer cell line), and mesothelial cells. The nitric oxide can then interact with O2 to form peroxynitrate (NOOO), which is a potent oxidizing compound (Choe, 1998; Thomas et al., 1994); although precise mechanisms leading to lung injury are uncertain. Asbestos has been found to be active in signal transduction pathways in lung epithelial cells and mesothelial cells important for fibrosis or malignant transformation (Yanssen et al., 1995). Numerous cell membrane-mediated signal transduction pathways are regulated by activation of phospholipase C or D, resulting to increased intracellular calcium and increased protein kinase C (PKC) activity. PKC translocates to the cell membrane when activated and mediates numerous cellular functions, including regulation of gene expression, cellular growth, cellular shape, and epithelial permeability (Winter et al., 1990). Asbestos has also been shown to activate tyrosine kinases, which promote cell proliferation, growth factor signal transduction, and alterations in cell–cell adherence (Christe, 2009; Kramp, 2009; Solbes and Harper, 2018). The proposed mechanisms outlining the major responses initiated by asbestos fibres exposure in the lungs tissue are shown in Figure 1. Similarly, asbestos exposure is associated with activation of oncogenes and loss of tumour suppressor genes (Solbes and Harper, 2018). Oncogenes play a central role in cell proliferation and cell transformation.
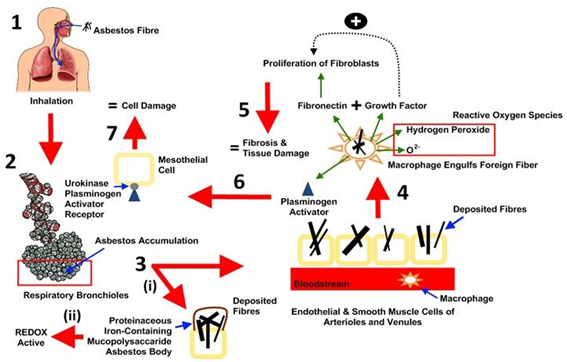
DISEASES ASSOCIATED TO ASBESTOS EXPOSURE
Depending on the level and duration of exposure, inhalation of asbestos fibers can cause different diseases such as asbestosis, malignant mesothelioma and lung carcinoma (ATSDR, 2016).
Asbestosis
Asbestosis is a diffuse pulmonary fibrosis caused by the inhalation of excessive amounts of asbestos fibres. It is a chronic and irreversible non-malignant asbestos-related disease in which symptoms typically start to develop several decades after exposure; these often progress to seriously affect normal daily activity and can lead to various complications which can be fatal (HSE, 2018). Asbestosis typically occurs in individuals with prolonged and heavy exposure to asbestos, and the disease may progress even after exposure has ceased. The diagnosis of asbestosis is usually based on the exposure history, clinical findings, and radiographic features. Asbestos fibres inhaled deep into the lung become lodged in the tissue, eventually resulting in diffuse alveolar and interstitial fibrosis. This fibrosis, according to Khan et al. (2004) can lead to reduced lung volumes, decreased lung compliance, impaired gas exchange, restrictive pattern of impairment, etc. Patients with asbestosis may have elevated levels of antinuclear antibody and rheumatoid factors and a progressive decrease in total lymphocyte count with advancing fibrosis (ATSDR, 2016).
Mesothelioma
Mesothelioma is a malignant cancer that principally affects the pleura and the peritoneum, which takes many years to develop following the inhalation of asbestos fibres. Its symptoms are typically non-specific and appear late in the development of the disease – becoming almost always fatal, and often within twelve months of symptom onset (HSE, 2018; Karen, 2018). Mesothelioma has been reported to have a strong relationship with asbestos that annual cases give a particularly clear view of the effect of past exposures, and as the disease is usually rapidly fatal following disease onset, the number of annual deaths closely approximates to the annual number of new cases (HSE, 2018). Pleural mesothelioma has been accounted for about 80%, while peritoneum mesothelioma accounts for about 20% of mesothelioma cases (Khan et al., 2013). Patients with malignant pleural mesothelioma can have sudden onset of pleural effusion and/or pleural thickening, dyspnea, and chest pain. The risk of mesothelioma depends on the amount and duration of asbestos exposure (CCOSH, 2018) and all types of asbestos can cause mesothelioma, but some researchers believe that the amphibole form is more likely to induce mesothelioma than the serpentine form (ATSDR, 2001; 2016). There has been rapid increase in annual deaths in Britain over the last 50 years, as a consequence of mainly occupational asbestos exposures that occurred because of the widespread industrial use of asbestos during 1950-1980 (HSE, 2018).
Table 2. Predicted 15-year cumulative mortality arising from mesothelioma (1994-2008) in some developing countries using asbestos but not reporting mesothelioma frequency
Adapted from Park et al. (2011) |
Lung Carcinoma
After tobacco, asbestos is one of the most common causes of lung cancer, which usually has no specific clinical signs associated with particular causes and so it is very difficult to be sure about the causes of individual cases. However, the overall proportion of annual deaths that are attributable to past asbestos exposures can be estimated from epidemiological information. Lung cancer is still typically fatal within a few years of diagnosis and so, as with the mesothelioma, the number of annual deaths is similar to the annual incidence of new cases (HSE, 2018). According to the 2004 Surgeon General Report on smoking, men with a lifetime of smoking have a 16 percent higher risk of dying from lung cancer over their lifetimes than those who do not smoke (IARC, 2012). It is estimated that 4-12% of lung cancers are related to occupational levels of exposure to asbestos (Henderson et al., 2004). Whether asbestos exposure will lead to lung cancer depends on several factors such as: level, duration, and frequency of asbestos exposure (cumulative exposure), time elapsed since exposure, age when exposure occurred, and of tobacco use (ATSDR, 2016; Henderson et al., 2004).
Epidemiological studies showed that of specific groups of workers that have heavy asbestos exposure in the past have typically estimated a greater number of lung cancers attributed to asbestos than there were mesotheliomas (McCormack et al., 2012). However, other studies that are more representative of the British population as a whole provide the best basis for estimating the overall number of asbestos-related lung cancers. Such evidences by Darnton et al. (2005) and Gilham et al. (2015) suggest that there are around as many lung cancer cases attributed to past asbestos exposure each year as there are mesotheliomas, though this estimate is uncertain.
An expectation by HSE (2018) is that there will be fewer asbestos-related lung cancers per mesothelioma in the future as a consequence of reductions in both asbestos exposure and smoking prevalence in past decades.
In recent years there have been (on average) around 275 new cases of asbestos-related lung cancer each year, with 180 reported in 2017, within the Industrial Injuries Disablement Benefit (IIDB) scheme and around 80 cases identified by chest physicians each year within the Health and Occupation Reporting (THOR) scheme, with 41 estimated cases in 2017 (HSE, 2018).
CONCLUSION
Nobody is completely safe from asbestos exposure and its associated dangers because everyone lives in a home, either goes to school or work, and lives in an urban or rural locations where measured concentrations of asbestos (low or high) is present. No matter how very low is the level of exposure, asbestos has been found to be dangerous to the human body.
References
- Agency for Toxic Substances and Disease Registry (ATSDR). (2001). Toxicological profile for asbestos. Atlanta GA: U.S. Department of Health and Human Services.
- Agency for Toxic Substances and Disease Registry (ATSDR). (2016). Case studies in environmental medicine: Asbestos Toxicity.
- American Thoracic Society. (2004). Diagnosis and initial management of nonmalignant diseases related to asbestos. American Journal of Respiratory and Critical Care Medicine, 170, 691-715. https://doi.org/10.1164/rccm.200310-1436ST
- Canadian Centre for Occupational Health and Safety. (2018). Occupational Safety and Health (OSH) Answers Fact Sheets: Asbestos Health Effects. Available at: https://www.ccohs.ca/oshanswers/chemicals/asbestos/effects.html (Accessed on November 10, 2018).
- Choe, N., Tanaka, S. and Kagan, E. (1998). Asbestos fibers and interleukin-1 upregulate the formation of reactive nitrogen species in rat pleural mesothelial cells. Am. J. Respir. Cell Mol. Biol., 19, 226–36. https://doi.org/10.1165/ajrcmb.19.2.3111
- Christe, M. (2009). Asbestos Risk Assessment. J. Ugrad. Boil. S. 1(4): 12-24.
- Cole, R. W., Ault, J. G., Hayden, J. H., et al. (1991). Crocidolite Asbestos Fibres Undergo Size Dependent Microtubule-Mediated Transport after Endocytosis in Vertebrate Lung Epithelial Cells. Cancer Res., 51, 4942–7.
- Darnton, A., McElvenny, D. and Hodgson, J. (2005). Estimating the number of asbestos related lung cancer deaths in Great Britain from 1980-2000. Annals of Occupational Hygiene, 50(1), 29-38. https://doi.org/10.1093/annhyg/mei067
- Encyclopedia of Chemical technology. (1948). New York and London, Interscience Publisher. (Accessed on September 23, 2018).
- Environmental Protection Agency (EPA). (2012a). Learn about asbestos. Washington DC. Available at: https://www.epa.gov/asbestos/learn-about-asbestos (Accessed: September 21, 2018).
- Gilham, C., Rake, C., Burdett, G., et al. (2015). Pleural mesothelioma and lung cancer risks in relation to occupational history and asbestos lung burden. Occup Environ Med., 73(5), 290-9. https://doi.org/10.1136/oemed-2015-103074
- Health and Safety Executive. (2018). Asbestos-related diseases in Great Britain, 2018.
- Henderson, D. W, Rodelsperger, K, Woitowitz, H. J. and Leigh, J. (2004). After Helsinki: a multidisciplinary review of the relationship between asbestos exposure and lung cancer, with emphasis on studies published during 19972004. Pathology, 36(6), 517-550. https://doi.org/10.1080/00313020400010955
- International Agency for Research on Cancer (IARC). (2012). Asbestos (chrysotile, amosite, crocidolite, tremolite, actinolite, and anthophyllite). IARC Monogr Eval Carcinog Risks Hum., 100C, 219–309.
- Janssen, Y. M., Heintz, N. H. and Mossman, B. T. (1995). Induction of c-fos and c-jun Protooncogene Expression by Asbestos is ameliorated by N-acetyl-L-cysteine in Mesothelial Cells. Cancer Res., 55, 2085–9.
- Kamp, D. W. (2009). Subspecialty in Translational Medicine: Asbestos-induced lung diseases: an update. Translational Research, 153(4), 143–152. https://doi.org/10.1016/j.trsl.2009.01.004
- Karen, S. R. N. (2018). What are the most common Mesothelioma Symptoms? Available at: https://www.asbestos.com/mesothelioma/symptoms/
- Khan, A., Irion, K., Ghanem, S., MacDonald, S. and Allen, C. M. (2004). Asbestos-related disease imaging. New York, NY.
- McCormack, V., Peto, J., Byrnes, G., et al. (2012). Estimating the Asbestos-Related Lung Cancer Burden from Mesothelioma Mortality. Br J Cancer, 106(3), 575-84. https://doi.org/10.1038/bjc.2011.563
- Nagoya, J. (2009). Mechanisms of Asbestos-Induced Carcinogenesis. Med. Sci., 71, 1-10.
- National Institute of Occupational Safety and Health (NIOSH). (2011a). Asbestos fibers and other elongate mineral particles: state of the science and roadmap for research. Current Intelligence Bulletin, 62. Publication Number 2011-159.
- National Toxicology Program (NTP). (2005). NTP 11th Report on Carcinogens. Rep. Carcinog. 111-A32. PMID: 1982656.
- NIH. (2009). Asbestos Exposure and Cancer Risk: National Cancer Institute. Available at: www.cancer.gov
- Rice, C. and Heinema, E. F. (2003). An asbestos ob exposurematrix to characterize fibre type, length, and relative exposure density. Appl Occup Environ Hyg, 18, 506-512. https://doi.org/10.1080/10473220301459
- Roberta, C. B. (2004). Asbestos, its Chemical and physical properties. Available at: https://environmentalchemistry.com/yogi/environmental/asbestosproperties2004.html (Accessed on September 18, 2018).
- Sekido, Y. (2013). Molecular pathogenesis of malignant mesothelioma. Carcinogenesis; 34, 1413–9. https://doi.org/10.1093/carcin/bgt166
- Solbes, E. and Harper, R. W. (2018). Biological responses to asbestos inhalation and pathogenesis of asbestos-related benign and malignant disease. J. Investig. Med., 66, 721–727. https://doi.org/10.1136/jim-2017-000628
- Steele, M. (2011). Asbestosis SD, Fibrosis A-IP. In: M Schwarz, T King, Interstitial Lung Disease (5th ed.). USA: People’s Medical Publishing House, 543–55.
- Thomas, G., Ando, T., Verma, K., et al. (1994). Asbestos Fibers and Interferon-Gamma Up-regulate Nitric Oxide Production in Rat Alveolar Macrophages. Am J Respir Cell Mol Biol., 1, 707–15. https://doi.org/10.1165/ajrcmb.11.6.7524571
- Toxicological Profile for Asbestos (2016). Agency for Toxic Substances and Disease Registry. Available at: www.atsdr.cdc.gov
- USGS. (2001). Some Facts about Asbestos (USGS Fact Sheet FS-012-01), 4pp.
- Weill, H., Hughes, J. M. and Churg, A. M. (2004). Changing trends in U.S. mesothelioma incidence. Occupational Environmental Medicine, 61, 438-41. https://doi.org/10.1136/oem.2003.010165
- WHO. (2006). Elimination of asbestos related diseases. WHO/SDE/OEH/06.03. Geneva: World Health Organization.
- WHO. (2014). Chrysolite Asbestos.
- Wright, J. L., Cagle, P., Churg, A., et al. (1992). Diseases of the small airways. Am. Rev. Respir. Dis., 146, 240–62. https://doi.org/10.1164/ajrccm/146.1.240
How to cite this article
Vancouver
Zephaniah HS, Aremu SO. Asbestos: A Silent Potent Killer. EUR J ENV PUBLIC HLT. 2019;3(2):em0030. https://doi.org/10.29333/ejeph/5906
APA
Zephaniah, H. S., & Aremu, S. O. (2019). Asbestos: A Silent Potent Killer. European Journal of Environment and Public Health, 3(2), em0030. https://doi.org/10.29333/ejeph/5906
AMA
Zephaniah HS, Aremu SO. Asbestos: A Silent Potent Killer. EUR J ENV PUBLIC HLT. 2019;3(2), em0030. https://doi.org/10.29333/ejeph/5906
Chicago
Zephaniah, Hannaniah Shenia, and Stephen Olaide Aremu. "Asbestos: A Silent Potent Killer". European Journal of Environment and Public Health 2019 3 no. 2 (2019): em0030. https://doi.org/10.29333/ejeph/5906
Harvard
Zephaniah, H. S., and Aremu, S. O. (2019). Asbestos: A Silent Potent Killer. European Journal of Environment and Public Health, 3(2), em0030. https://doi.org/10.29333/ejeph/5906
MLA
Zephaniah, Hannaniah Shenia et al. "Asbestos: A Silent Potent Killer". European Journal of Environment and Public Health, vol. 3, no. 2, 2019, em0030. https://doi.org/10.29333/ejeph/5906